As the global energy system rapidly shifts toward decarbonization and electrification, grid operators face new challenges in maintaining system reliability. Increasing reliance on renewable energy introduces variability and intermittency that conventional grids were never designed to handle.
Battery Energy Storage Systems (BESS) have emerged as a flexible, fast-responding solution that supports both reliability and the seamless integration of renewables. From frequency regulation to emergency backup, BESS is playing a critical role in transforming how we build and operate the modern power grid.
This article explores how BESS enhances grid stability, enables renewable adoption, and shapes the future of power systems.
The Grid Reliability Challenge
As the global power system undergoes one of the most significant transitions in its history, maintaining grid reliability has become an increasingly complex and urgent challenge. The traditional power grid, built for centralized, predictable, fossil-based generation, is now being stretched beyond its original design limits by the rise of renewables, distributed energy resources (DERs), and evolving consumer demands. Most of the existing transmission and distribution infrastructure was designed decades ago, built around a model in which a small number of large, dispatchable power plants generated electricity and delivered it in one direction to end users. This unidirectional, top-down structure made control and coordination relatively straightforward.
Today, however, the grid must accommodate a radically different energy ecosystem characterized by:
- A surge in distributed generation: Rooftop solar panels, community wind projects, and customer-sited battery storage systems are injecting power at the grid’s edge, often unpredictably and with minimal central oversight.
- Electrification of end-use sectors: The proliferation of electric vehicles (EVs), heat pumps, and all-electric buildings is increasing overall demand while also introducing new, highly variable load patterns.
- Bidirectional power flows: With DERs exporting power to the grid, electricity now flows in multiple directions, requiring more sophisticated control systems and visibility across the network.
- Digital demand response and real-time market dynamics: Smart appliances, automated building systems, and responsive industrial loads add further variability that grid operators must factor into their planning and operations.
These trends are exposing the limitations of legacy infrastructure, which was never designed to manage such dynamic, decentralized, and intermittent sources of energy. As a result, grid operators now face unprecedented challenges in balancing supply and demand, ensuring voltage and frequency stability, and managing transmission congestion, all in real time.
The Need for Flexibility
Grid reliability hinges on a delicate, second-by-second balance between electricity generation and consumption. Even minor imbalances can cause frequency or voltage deviations, risking equipment damage, system instability, or widespread outages. Historically, this balance has been maintained using slow-ramping fossil fuel plants, such as gas peakers or coal units operating as spinning reserves.
However, these traditional resources lack the speed, agility, and scalability needed to respond to the rapid fluctuations introduced by high levels of renewable generation, especially solar and wind, which can vary minute to minute based on weather conditions.
This is where Battery Energy Storage Systems (BESS) play a transformative role. Unlike conventional generation, BESS can:
- React in milliseconds to frequency deviations, absorbing or injecting power as needed.
- Stabilize voltage by providing or absorbing reactive power through smart inverters.
- Shift load by storing energy when it’s abundant (and cheap) and discharging when it’s scarce (and expensive).
- Provide emergency backup power and even initiate black start procedures to re-energize substations during system outages.
By enabling flexibility at both the transmission and distribution levels, BESS acts as a shock absorber for the grid, managing volatility, supporting ramping requirements, and improving the overall stability of increasingly complex energy networks.
Strategic Implications
The grid reliability challenge is not just a technical issue, it’s a strategic imperative for utilities, regulators, and energy developers. Without modern tools to manage variability, the energy transition risks being slowed by reliability concerns, infrastructure bottlenecks, and increased costs. BESS addresses this gap by providing fast, modular, and scalable flexibility, unlocking the ability to integrate more renewables without sacrificing reliability or resilience.
As grid operators move from generation-following-load to load-following-generation, the role of storage becomes even more vital, bridging the gap between clean energy ambitions and real-time grid stability.
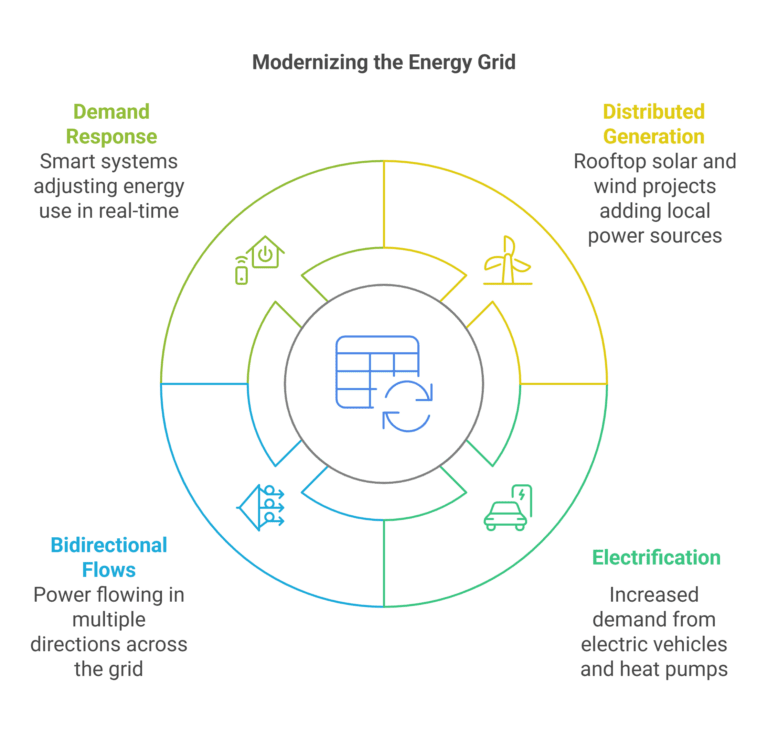
How BESS Supports Grid Reliability
Battery Energy Storage Systems (BESS) are increasingly recognized as indispensable assets for grid operators seeking to maintain reliable, stable, and resilient power systems. As the energy mix becomes more decentralized and renewable-heavy, BESS plays a multifaceted role in addressing key reliability challenges.
Frequency Regulation
Electric grids must maintain a stable frequency, typically 60 Hz in North America and 50 Hz in many other regions, to ensure synchronous operation of all equipment connected to the system. Frequency deviations, even minor ones, can lead to equipment malfunction, grid instability, or cascading blackouts. Traditionally, frequency regulation has relied on spinning reserves, large generators operating below capacity, ready to ramp up or down.
BESS provides a far superior alternative. It can detect frequency deviations in real time and respond in milliseconds, either injecting power into the grid during under-frequency events or absorbing power during over-frequency conditions. This ultra-fast response enables fine-tuned balancing without the need for fuel-based generation to stay online.
Key benefits include:
- Participation in ancillary services markets: BESS can provide Frequency Regulation and Fast Frequency Response (FFR) services, earning revenue while stabilizing the grid.
- Reduced carbon footprint: Fast-response energy storage reduces the need for fossil-fueled peaker plants or spinning reserves.
- Higher accuracy: Digital controls allow precise response within narrow frequency tolerances.
Voltage Support and Reactive Power Compensation
Voltage stability is just as critical as frequency. Variations in voltage can lead to overheating, motor inefficiencies, or even large-scale equipment failures. Maintaining optimal voltage levels across the grid, especially at the distribution level, is increasingly difficult with rising penetration of inverter-based renewable resources like solar PV.
Modern BESS installations often include advanced inverters capable of providing reactive power independently of real power delivery. These inverters can quickly respond to local voltage drops or spikes, supporting voltage profiles and maintaining power factor.
Key capabilities include:
- Volt-VAR control: Automated adjustment of reactive power to maintain voltage setpoints.
- Grid-forming inverters: Allowing the BESS to “set” voltage and frequency in weak or islanded systems.
- Deferral of infrastructure upgrades: Reducing or eliminating the need for new capacitor banks, voltage regulators, or substation enhancements.
Example: In California, BESS systems deployed in areas with high rooftop solar penetration are used to regulate voltage swings during mid-day generation peaks and evening load ramps, helping utilities avoid costly hardware upgrades.
Peak Shaving and Load Shifting
The electric load on a grid fluctuates daily, with demand typically peaking in the late afternoon or early evening. These demand peaks drive the need for expensive peaker plants, which are often inefficient and carbon-intensive. BESS offers a flexible, scalable solution to this challenge through peak shaving and load shifting.
BESS charges during off-peak periods when electricity is cheaper and demand is low, then discharges during peak hours to reduce load on the grid. This helps:
- Avoid demand charges for large commercial and industrial customers.
- Reduce system-wide peak loads, deferring investment in new generation capacity.
- Support renewables by absorbing excess solar or wind during generation peaks and releasing it later.
Example: A 100 MW / 400 MWh BESS in New York can reduce demand during summer heatwaves, replacing the need for aging gas-fired peaker units and supporting clean energy goals.
Black Start Capabilities
During a complete grid outage (blackout), most conventional power plants cannot restart on their own, as they require external power for systems like pumps, controls, and excitation. BESS can provide the initial energy to restore power at substations, energize transmission lines, and “jump-start” larger thermal or hydroelectric units—a process known as black start.
Advantages include:
- Fast recovery from outages with minimal reliance on diesel generators.
- Improved resilience in the face of natural disasters, cyberattacks, or equipment failure.
- Support for grid restoration protocols defined by ISOs and utilities.
Example: In Texas, a utility-integrated BESS has demonstrated black start capabilities by successfully re-energizing substations and restoring power without traditional black start units.
Microgrid and Islanding Support
A microgrid is a localized group of interconnected loads and distributed energy resources that can operate in conjunction with or independently from the main grid. BESS is essential for microgrids because it:
- Balances generation and demand in real time, enabling seamless transition between grid-connected and islanded modes.
- Provides frequency and voltage regulation within the microgrid.
- Supports critical loads during outages, making microgrids viable for high-reliability applications.
This capability is invaluable for:
- Remote communities disconnected from centralized power infrastructure.
- Critical facilities like hospitals, data centers, military bases, and emergency shelters.
- Commercial campuses or industrial parks seeking energy autonomy and resilience.
Example: The Stone Edge Farm microgrid in California successfully islanded during regional wildfires, using BESS to coordinate energy flows between solar, fuel cells, and loads for uninterrupted operation.
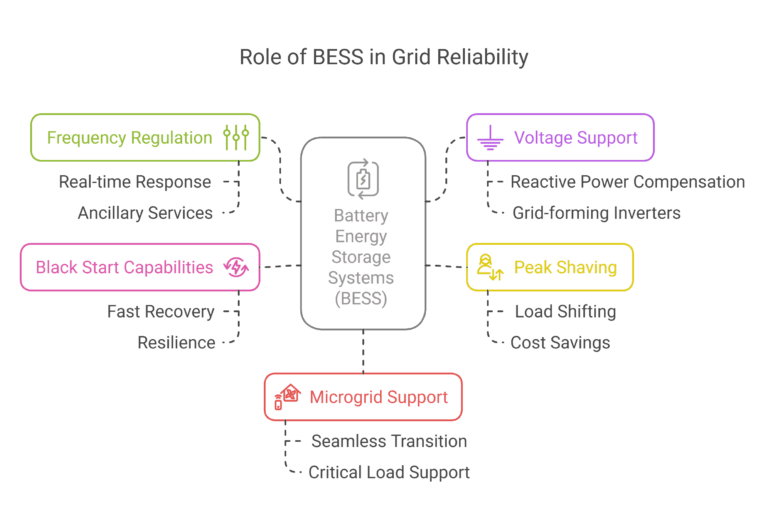
Integrating Renewables with BESS
As the global energy landscape shifts toward high renewable penetration, the integration of intermittent resources like wind and solar presents operational and planning challenges for grid operators. Battery Energy Storage Systems (BESS) act as a critical enabler for addressing these challenges by improving dispatchability, smoothing power output, and supporting grid stability. BESS is not merely a complementary asset, it’s a strategic cornerstone for the next-generation power system.
Smoothing Output and Managing Curtailment
Solar and wind energy are inherently non-dispatchable—they produce electricity only when the sun shines or the wind blows. This intermittency creates steep ramp-up and ramp-down events, known as the “duck curve” in solar-dominant regions. Grid operators often resort to curtailment, shutting down otherwise available clean power, or rely on fast-start fossil fuel generators to manage these fluctuations, both of which are suboptimal from an economic and environmental standpoint.
BESS provides a buffer between generation and demand by:
- Capturing excess energy during times of overproduction (e.g., mid-day solar peaks).
- Releasing energy during low-production or high-demand periods (e.g., after sunset or during calm weather for wind).
- Smoothing ramp rates, allowing renewable generation to mimic the controlled behavior of traditional plants.
This functionality reduces the need for curtailment, enhances asset utilization, and allows renewable power to contribute more consistently to grid stability.
Example: In California’s CAISO territory, BESS has been used to reduce solar curtailment during spring afternoons when generation exceeds demand. Storage shifts this excess into the evening peak, supporting both reliability and decarbonization.
Co-Located Systems
Co-locating BESS with renewable generation, typically referred to as a hybrid renewable-storage plant, is becoming a standard project development model. In this configuration, both systems share infrastructure, permitting, and grid interconnection points, significantly reducing capital expenditures and deployment timelines.
Key advantages of co-located systems include:
- Shared Interconnection: One point of interconnection simplifies permitting and reduces costs.
- Improved Dispatchability: The hybrid system can supply firm or time-shifted power, reducing grid variability.
- Financial Optimization: Battery charging with on-site renewable energy can qualify for Investment Tax Credits (ITC) under U.S. policy, and other global incentives.
- Higher Capacity Factor: The ability to store and dispatch clean energy during non-generation hours boosts the effective utilization of the system.
Example: The Slate project in California (300 MW solar + 140.25 MW / 561 MWh BESS) is designed to provide around-the-clock renewable power to corporate offtakers, meeting demand peaks and offering ancillary services to CAISO.
Virtual Power Plants (VPPs)
A Virtual Power Plant (VPP) is a digital aggregation of decentralized energy resources, such as rooftop solar, behind-the-meter batteries, electric vehicles (EVs), and demand-responsive loads, that operate in coordination as a unified resource. BESS plays a pivotal role in VPPs by enabling real-time energy balancing and providing fast-responding grid services.
With intelligent software and predictive analytics, VPPs can:
- Provide frequency response, spinning reserve, and voltage regulation to grid operators.
- Respond to real-time market signals, enabling participation in wholesale markets or demand response programs.
- Alleviate local grid congestion, allowing utilities to defer transmission and distribution (T&D) upgrades.
- Enhance resilience by isolating and supporting critical loads during outages or grid stress events.
This model is particularly attractive for utilities facing high DER (distributed energy resource) penetration or those looking to modernize grid operations without major infrastructure investments.
Example: Tesla’s South Australia VPP connects thousands of residential Powerwalls into a coordinated network, delivering ancillary services and helping stabilize the grid. During an outage event in 2021, the VPP responded within milliseconds to balance frequency and prevent widespread blackouts.
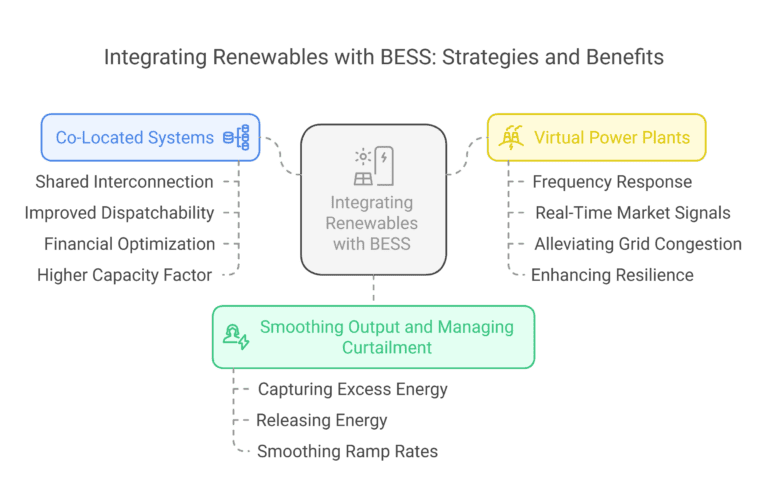
Summary of BESS-Renewable Integration Benefits
Capability | Benefit | Stakeholders Impacted |
Output Smoothing | More predictable and dispatchable renewable output | Grid operators, asset owners |
Curtailment Reduction | Higher utilization of renewable generation | Project developers, offtakers |
Hybrid Configurations | Lower costs, improved economics, better ROI | IPPs, utilities, investors |
VPP Enablement | Grid services, DER monetization, enhanced resilience | Aggregators, utilities, end-users |
Market Participation | Flexibility to engage in energy, capacity, and ancillary markets | Energy traders, system planners |
Market and Economic Drivers
Battery Energy Storage Systems (BESS) are rapidly transitioning from pilot-scale applications to mainstream infrastructure, propelled by strong market forces and policy mechanisms. The convergence of declining costs, favorable regulations, and diversified revenue opportunities is reshaping the economic landscape for storage deployment across all scales.
Declining Costs
One of the most significant catalysts for BESS deployment is the dramatic reduction in system costs over the past decade. Lithium-ion battery prices have dropped by over 80% since 2010, driven by:
- Advances in battery chemistry (e.g., LFP for safety and longevity, NMC for energy density).
- Gigafactory-scale manufacturing in Asia, Europe, and North America.
- Economies of scale and vertical integration across supply chains.
- Process improvements in thermal management, packaging, and energy density.
As costs continue to fall, other chemistries are emerging to serve niche applications:
- Sodium-ion offers promise for non-lithium alternatives, especially in markets sensitive to resource availability.
- Flow batteries (e.g., vanadium redox) offer long-duration storage with minimal degradation, ideal for grid balancing over 6+ hours.
These cost trends are opening new frontiers for BESS in residential, commercial & industrial (C&I), and utility-scale markets, with total system cost targets now approaching competitive parity with conventional generation and grid solutions.
Policy Incentives
Government support plays a pivotal role in accelerating BESS adoption, particularly in early or transitional markets. Several key policy mechanisms are shaping deployment trends:
- Federal Tax Incentives: The Inflation Reduction Act (IRA) extends the Investment Tax Credit (ITC) to standalone storage, offering up to 30% credit for eligible projects.
- State-Level Mandates: States like California (SB 100) and New York (CLCPA) have mandated aggressive clean energy and storage deployment targets.
- Federal Regulatory Support: FERC Orders 841 and 2222 have redefined market rules, enabling storage and aggregated DERs to access wholesale revenue streams.
These policies reduce project risk, improve return on investment, and attract private capital, particularly for developers seeking to scale projects rapidly.
Revenue Stacking
To enhance economic viability, modern BESS systems are designed to pursue multiple revenue streams simultaneously, a strategy known as revenue stacking. This is enabled by sophisticated control systems and market participation models, allowing storage to dynamically switch between services based on grid needs and price signals.
Key value streams include:
- Energy Arbitrage: Buy electricity during off-peak hours and sell during peak pricing windows.
- Capacity Market Participation: Offer firm capacity to ensure system reliability.
- Ancillary Services: Provide fast frequency response, spinning reserve, voltage support, and black start services.
- Demand Charge Reduction: Lower utility bills for behind-the-meter (BTM) customers by shaving peak usage.
- Grid Services: Alleviate congestion, defer upgrades, and provide locational value to utilities.
This flexibility not only boosts financial returns but also enhances grid resilience and supports broader decarbonization goals.
Project Development Considerations
Successful deployment of BESS projects requires careful navigation of technical, regulatory, and operational factors. Developers must address site suitability, permitting, integration, and stakeholder alignment throughout the project lifecycle.
Siting and Interconnection
Choosing the right location is critical for maximizing both system performance and project economics. Key considerations include:
- Proximity to load centers or renewable generation sources.
- Available interconnection capacity and minimal grid congestion.
- Environmental sensitivities, such as wetlands, endangered species habitats, or proximity to urban developments.
- Permitting complexity and local stakeholder acceptance.
Interconnection remains one of the largest barriers to BESS deployment, with lengthy queues, high upgrade costs, and evolving standards. Developers must engage early with transmission operators and utilities to de-risk timelines and budgets.
Safety and Standards
As BESS capacity scales, safety remains a primary concern for regulators, insurers, first responders, and the public. Comprehensive safety planning includes:
- Compliance with NFPA 855: The primary fire code for energy storage installation.
- UL 9540 / UL 9540A: Certification and thermal runaway testing to validate system safety under stress conditions.
- Emergency response coordination: Clear fire suppression, ventilation, and access strategies.
Proper enclosure design, thermal management, and system-level fault protection (e.g., DC disconnects, fire barriers) are essential to mitigate the risk of catastrophic failure or cascading events.
EPC and Commissioning
BESS projects demand tight coordination across multiple vendors, technologies, and subsystems. The engineering, procurement, and construction (EPC) phase must address:
- Integration of batteries, PCS (Power Conversion Systems), and EMS (Energy Management Systems).
- Site-specific civil and electrical work, including transformers, protection systems, and HVAC.
- SCADA and communications setup, ensuring reliable data flow for operations and market bidding.
Commissioning is a multi-phase process that includes:
- Functional testing of control logic and grid dispatch commands.
- Validation of fault response, islanding detection, and re-synchronization.
- Grid integration testing, especially for systems providing services to ISOs or RTOs.
Successful commissioning requires a detailed plan, vendor collaboration, and rigorous quality assurance.
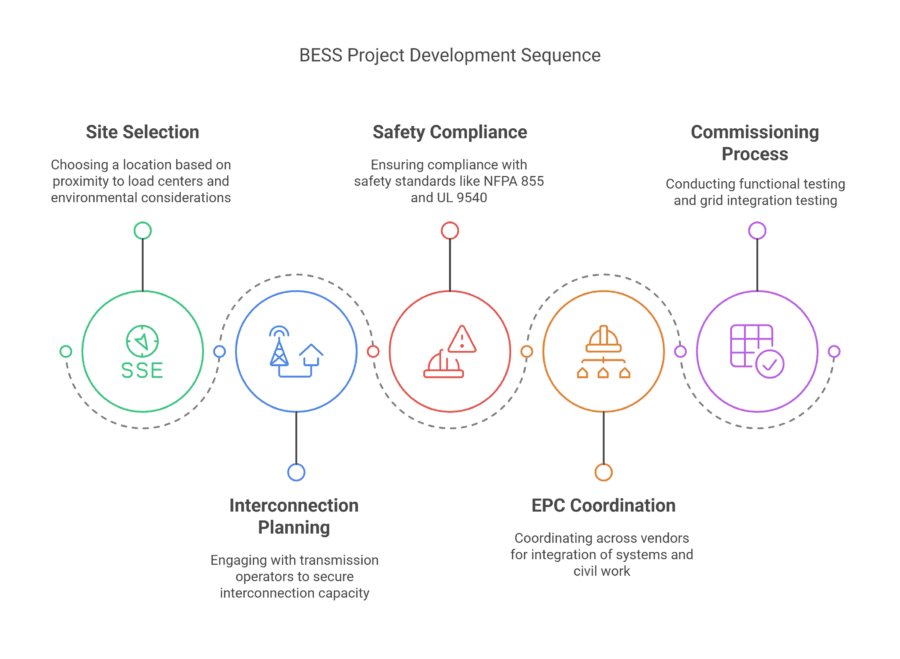
Regulatory Environment
The regulatory framework governing BESS continues to evolve as grid operators and policymakers recognize the value of storage in transitioning to a more dynamic, decentralized, and decarbonized power system.
Federal Framework
Two landmark FERC orders have fundamentally reshaped the role of storage in wholesale markets:
- FERC Order 841 (2018): Requires regional transmission operators (RTOs) and independent system operators (ISOs) to allow energy storage to participate in energy, capacity, and ancillary services markets.
- FERC Order 2222 (2020): Enables the aggregation of distributed energy resources (DERs), including BESS, to act as market participants.
These orders facilitate market access, clarify dispatch rights, and improve price signal visibility, paving the way for a more level playing field between centralized and decentralized resources.
State-Level Programs
States are leading the charge in developing innovative BESS policies. Examples include:
- California (CPUC): Targets 5 GW of storage by 2030, supported by procurement mandates, SGIP rebates, and emergency reliability contracts.
- New York (NYSERDA, PSC): Aims for 6 GW of energy storage by 2030 through incentive programs, clean energy standard revisions, and ConEd demand reduction pilots.
- Massachusetts: The Clean Peak Standard incentivizes storage to discharge during high-emissions periods, effectively rewarding time-shifted clean energy.
Each state’s regulatory and market conditions vary significantly, requiring developers to tailor their strategies by geography, utility territory, and service offering.
Challenges and Limitations
Despite strong momentum, BESS faces several challenges that could limit the pace and scale of adoption without targeted solutions.
Supply Chain and Raw Materials
BESS deployment is tightly linked to the global supply of critical minerals such as lithium, cobalt, nickel, and graphite. Current concerns include:
- Concentration of processing in specific countries (e.g., China dominates over 70% of global battery supply chain processing).
- Environmental and social concerns around mining practices, including child labor and habitat disruption.
- Price volatility and long lead times for procurement.
Industry efforts to address these challenges include:
- Recycling and circular economy models to recover valuable metals from retired batteries.
- Second-life batteries from electric vehicles repurposed for stationary applications.
- Domestic mining and processing initiatives, backed by U.S. DOE and other national agencies.
Lifecycle Degradation
Battery performance degrades over time due to chemical and mechanical stress. Key drivers of degradation include:
- Depth of discharge (DoD): Higher DoD accelerates wear.
- Charge/discharge cycles and rate: Frequent or rapid cycling stresses battery cells.
- Thermal conditions: Extreme temperatures reduce lifespan and safety.
To manage these risks, developers rely on:
- Battery Management Systems (BMS) that monitor voltage, temperature, and charge balance in real time.
- Warranty planning with OEMs for performance guarantees over 10–15 years.
- Oversizing strategies to account for capacity fade and ensure contract compliance.
Integration Complexity
Integrating BESS into the power system is not a plug-and-play process. Complexities arise from:
- Interfacing with utility SCADA and protection systems to ensure safe operation.
- Participation in dynamic energy markets, which requires fast, accurate communication and forecasting.
- Cybersecurity risks due to increasing digital control and remote operation.
Successful integration demands:
- Robust testing and simulation, including hardware-in-the-loop (HIL) verification.
- Vendor interoperability and modular design, allowing components to evolve without full system overhauls.
- Ongoing operations and maintenance (O&M) plans, including software updates and performance monitoring.
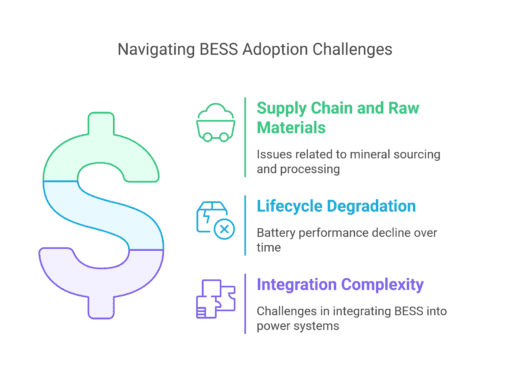
Future Trends
As energy systems evolve toward higher levels of renewable penetration, electrification, and decentralization, Battery Energy Storage Systems (BESS) are entering a new era of innovation. Future trends will be shaped not only by hardware breakthroughs but also by advancements in system integration, artificial intelligence, and grid architecture. These developments will expand the role of storage well beyond short-duration balancing and into the realms of long-duration reliability, deep decarbonization, and autonomous grid support.
Emerging Chemistries
While lithium-ion continues to dominate the storage landscape due to its proven performance, scalability, and falling costs, new chemistries are poised to diversify the market. These next-generation technologies aim to address the limitations of lithium-based systems, namely, cost, safety, raw material availability, and long-duration capabilities.
- Sodium-Ion Batteries
Sodium-ion technology uses more abundant and widely available materials, such as sodium and aluminum, making it attractive for regions lacking lithium or cobalt supply chains. Although energy density is lower compared to lithium-ion, sodium-ion offers:- Improved cost-effectiveness for short-duration grid applications.
- Enhanced thermal stability and safety, reducing fire risk.
- Potential for local manufacturing and reduced geopolitical dependency.
Companies like CATL and Natron Energy are already scaling sodium-ion for utility and commercial use, particularly in data centers and telecom backup systems.
- Flow Batteries
These systems store energy in liquid electrolytes that flow through electrochemical cells. They are ideally suited for 4–12 hour applications, offering:- Virtually unlimited cycle life.
- Independent scaling of power (cell stack) and energy (tank size).
- No degradation from deep cycling or long idle periods.
Flow batteries (e.g., vanadium redox, zinc-bromine) are gaining traction for use in remote grids, microgrids, and renewable smoothing applications.
- Solid-State Batteries
Replacing flammable liquid electrolytes with solid materials, solid-state batteries offer:- Higher energy density (potentially 2x lithium-ion).
- Improved safety due to lower thermal runaway risk.
- Faster charging and longer cycle life.
While still in early-stage commercialization, breakthroughs in materials (e.g., lithium-metal, sulfide-based conductors) are pushing this technology closer to market for EVs and eventually grid-scale storage.
These chemistries are expected to expand the application range of storage systems—from high-frequency cycling in urban centers to long-duration dispatch in rural and remote areas—and reduce dependency on constrained materials like lithium, cobalt, and nickel.
Long-Duration Storage (LDS)
To fully enable a net-zero electricity system, multi-day to seasonal storage solutions will be required to bridge gaps in renewable generation during prolonged periods of low solar or wind output, what’s often referred to as “dunkelflaute” in European planning circles.
Key LDS technologies include:
- Pumped Hydro Energy Storage (PHES)
The most mature form of long-duration storage, PHES provides massive capacity (100s of MW to GW-scale) and durations of 6–24+ hours. Although geographically limited, new "closed-loop" pumped hydro projects are under evaluation in previously unutilized areas. - Compressed Air Energy Storage (CAES)
These systems store energy by compressing air in underground caverns or pressure vessels and then releasing it to spin a turbine. While less efficient than BESS, CAES can provide grid-scale inertia and multi-day storage with proper thermal management. - Hydrogen and Power-to-X
Excess renewable energy can be used to produce green hydrogen via electrolysis. Hydrogen can then be stored and converted back into electricity through fuel cells or turbines, or used in industrial processes. Hydrogen offers:- Seasonal energy storage potential.
- Cross-sector decarbonization (e.g., transport, industry).
- Scalability through existing gas infrastructure.
- Thermal Storage Systems
These technologies store energy as heat (e.g., molten salts, phase-change materials) and convert it back to electricity or use it for industrial heating. Emerging models couple solar or wind with high-temperature storage for on-demand dispatch or cogeneration.
While BESS remains the optimal choice for fast-response, short-duration applications, LDS is essential for ensuring reliability across time scales, particularly in regions pursuing 80%–100% renewable targets.
AI and Optimization
As BESS systems become more widely deployed and increasingly complex in operation, artificial intelligence (AI) and machine learning (ML) are emerging as key enablers for performance optimization, predictive control, and dynamic grid integration. These digital layers are transforming storage from static energy buffers into active, intelligent grid agents.
Key applications include:
- Predictive Maintenance and Asset Health Monitoring
AI models analyze operational data (voltage, temperature, state-of-charge, etc.) to detect anomalies, predict failures, and schedule maintenance proactively. This reduces downtime and extends asset life. - Price Forecasting and Market Bidding
Sophisticated algorithms ingest weather forecasts, market data, and grid conditions to optimize energy arbitrage, frequency response participation, and capacity commitments. This results in:- Maximized revenue capture from stacked value streams.
- Improved grid coordination during high-volatility events.
- Real-Time Optimization for Grid Support
AI can orchestrate fleets of distributed BESS assets to provide synthetic inertia, voltage support, and black start capabilities based on real-time grid conditions. This is particularly valuable for VPP operators, transmission planners, and utilities. - Autonomous Operation in Microgrids
AI-driven energy management systems can dynamically balance loads and generation within islanded or weak-grid environments—optimizing for resilience, cost, and emissions without manual input.
Example: Grid-edge software platforms from companies like AutoGrid, Fluence, and Wärtsilä use AI to manage gigawatts of storage and renewable assets globally, making decisions every few seconds based on evolving grid signals.
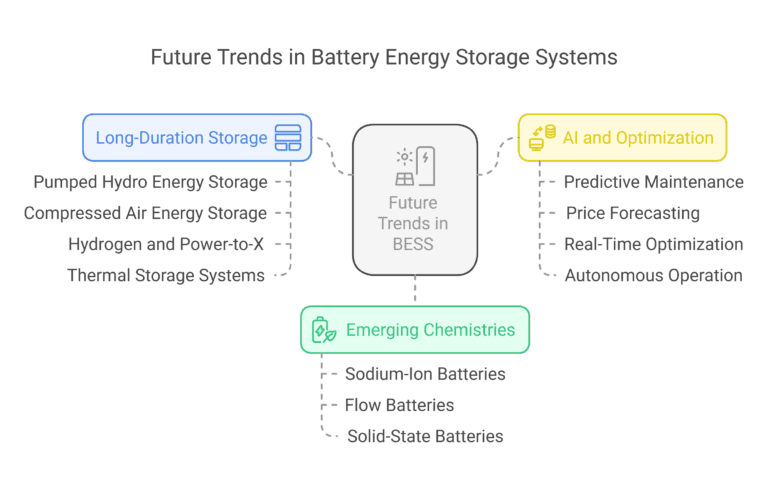
Summary of Future Trends
Trend | Key Features | Strategic Impact |
Emerging Chemistries | Sodium-ion, flow, solid-state | Broader application, lower costs, material diversification |
Long-Duration Storage | Multi-day to seasonal capacity | Grid decarbonization, reliability across time horizons |
AI and Digital Optimization | Predictive analytics, real-time control | Revenue maximization, operational efficiency, automation |
Conclusion
Battery Energy Storage Systems (BESS) are no longer a niche or experimental solution—they are rapidly becoming a cornerstone of modern grid infrastructure. Initially deployed to support limited-scale applications such as renewable smoothing or backup power, today’s BESS projects are central to solving the most complex challenges facing power systems: decarbonization, decentralization, and digitalization.
Thanks to their unmatched speed, modularity, and controllability, BESS can provide a wide array of services that were traditionally delivered by inflexible, fossil-fueled generation. Whether responding in milliseconds to frequency deviations, enabling peak demand reduction, or integrating with distributed and variable renewable energy sources, BESS is proving essential for ensuring both grid reliability and flexibility in a transforming energy landscape.
The evolution of BESS is being accelerated by several converging factors:
- Rapid cost declines in lithium-ion and alternative chemistries, lowering barriers to entry across all market segments.
- Regulatory shifts that open wholesale markets and reward flexibility, creating new monetization pathways.
- Advanced analytics and AI tools that turn static storage into active, grid-responsive assets.
- Public and private investment momentum, fueled by climate goals, electrification initiatives, and federal incentives like the Investment Tax Credit (ITC).
As we look ahead, BESS will be foundational to achieving a clean, resilient, and affordable energy future. It enables:
- High renewable penetration by smoothing intermittency and time-shifting supply.
- Grid resilience and modernization, offering black start capabilities, voltage control, and support for microgrids.
- Decentralized energy models, empowering consumers, communities, and commercial operators to actively participate in energy markets.
For utilities, this means rethinking grid planning and incorporating storage as a core asset class rather than an add-on. For developers and IPPs, it demands integrated approaches that combine storage with renewables, data intelligence, and flexible dispatch strategies. And for regulators and policymakers, it requires the continued evolution of market rules, interconnection standards, and incentive frameworks to fully unlock storage’s value.
Now is a critical inflection point. Thoughtful deployment strategies, standardized safety and performance protocols, and coordinated ecosystem collaboration will be essential to realize the full system-wide potential of energy storage. By embracing these priorities, stakeholders across the energy value chain can accelerate the transition to a grid that is not only cleaner and more efficient, but also more adaptive, resilient, and future-ready.
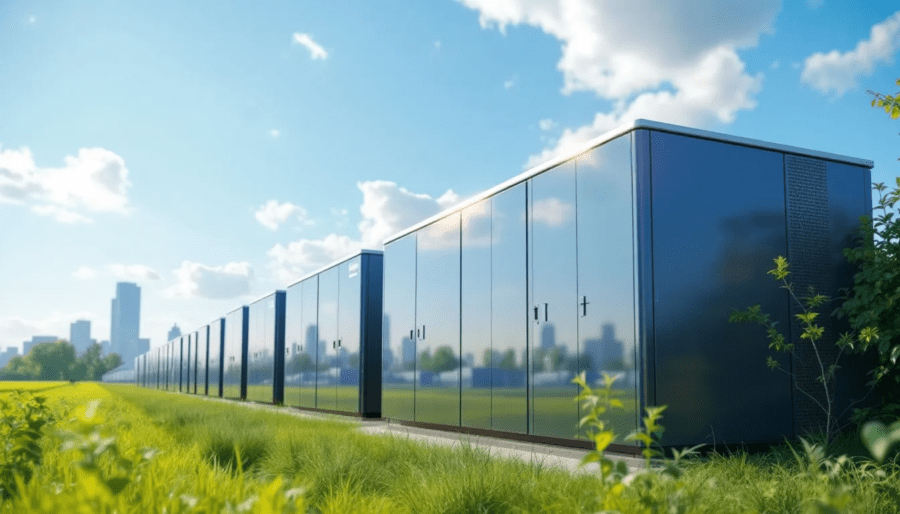
Disclaimer
The information provided in this post is for reference purposes only and is intended to serve as a guide to highlight key topics, considerations, and best practices. It does not constitute professional advice or a substitute for consulting regarding specific projects or circumstances. Readers are encouraged to evaluate their unique project needs and seek tailored advice where necessary. Please Contact Us to discuss your particular project.